Overview
Introduction
Coastal waters are dynamic and changing environments. Satellite-derived bathymetry (SDB) has been developed to survey water depths and other seabed characteristics by measuring the reflectance of the sea bottom extracted from the upwelling light signal.
Deriving bathymetry from satellite has key benefits being more cost effective, requiring no expensive field surveying, is available for remote and inaccessible areas and quicker to delivery than traditional methods.
ARGANS has developed innovative methodologies for generating IHO-compliant nautical charts derived from satellite multi-spectral images. The main drivers for IHO-compliant SDB are the need to achieve full coverage, a horizontal charting precision compatible with the ship’s own navigational precision, as provided by its current positioning system, and a reliable shallow danger alert.
Products
- Satellite Derived Bathymetry Models
- IHO-compliant nautical charts
- Water Properties
- Depth of Penetration
- Seabed Classification
Projects
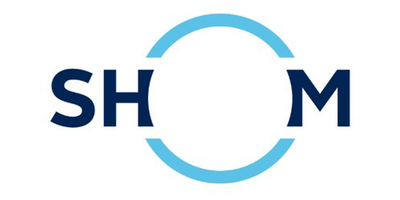
SHOM
The French hydrographic and oceanographic service (Service hydrographique et océanographique de la Marine) fund an experimental study into the use of Satellite Derived Bathymetry on a range of different locations with contrasting water quality. This project looks at the use of different sensors (spatial and sensor resolutions) for the application of satellite derived bathymetry.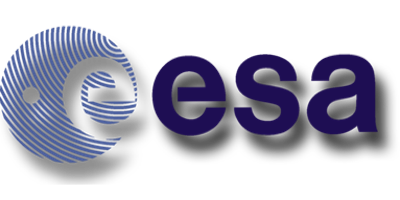
ESA
The European Space Agency (ESA) fund a promotional activity looking at the possible use of Sentinel 2 products in satellite-derived using a study over four different location.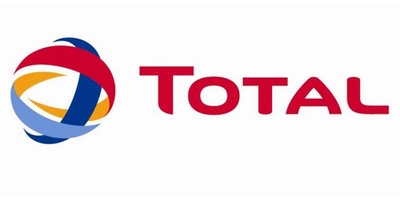
TOTAL
TOTAL S.A. the French multinational oil and gas company, has funded ARGANS on a number of occasions to provide satellite-derived bathymetry surveys of coast area and to to compare them with existing charts t0 promote avenues of innovation.Background
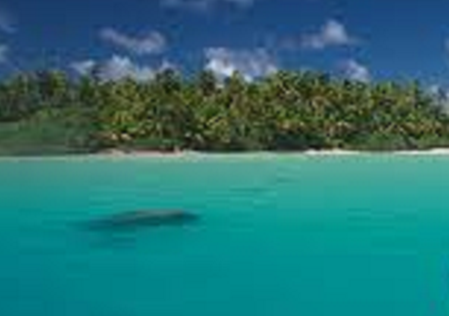
Historical Context
Careful visual observation along with lead line soundings have been, from ancient times, the Navigator’s best asset for survival from the dangers of shallow waters. Whether lookouts from the tops, later the crow's nest, or from the bridge, wearing Polaroid sunglasses; Mariners would watch for dark regions of the water that presaged danger of shoaling water over a coral pinnacle or rocks. A refinement of this visual approach was, during the Second World War, to estimate water depth from aerial photography, widely used by Government Agencies in field-proven topographic applications. In the nineteen eighties with the advent of improved satellite imagery brought satellite derived bathymetry (SDB).
Advantages of Satellite Derived Bathymetry
Using satellite remote sensing within a bathymetric mapping program potentially addresses these issues. Remote sensing should be seen as a complementary technique to in-situ field surveys. The two approaches produce different types of data which together can be used to build a picture of coastal bathymetric features across a wide range of spatial and temporal scales.
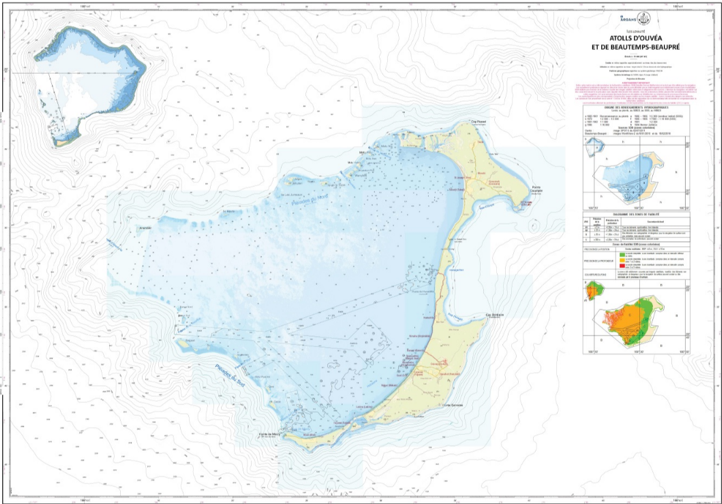
Nautical Charting
The first hydrographic attempt took place in the South Pacific with the first nautical chart of Ouvea, using a 1988 SPOT-1 image. Prior to 1986, satellite applications had been preceded by push-broom scanner simulations. Since its inception SDB has been widely used across a large spectrum of laboratories and private companies in Australia, Canada, the US and France amongst others.
Whilst academic & other private providers have focussed on environmental mapping, ARGANS, due to its early hydrographic core-business, concentrated on nautical charting applications, with a view to achieve strict compliancy with the IHO S-44 survey standards and cartographers concern regarding Quality Control and Safety of Navigation.
Data Acquisition
We can provide very high (2m) resolution using DigitalGlobe WorldView / CNES Pléiades imagery, or low resolution using US Landsat, but our primary image source is the European Commission Copernicus Sentinel-2 Multispectral Instrument (MSI).
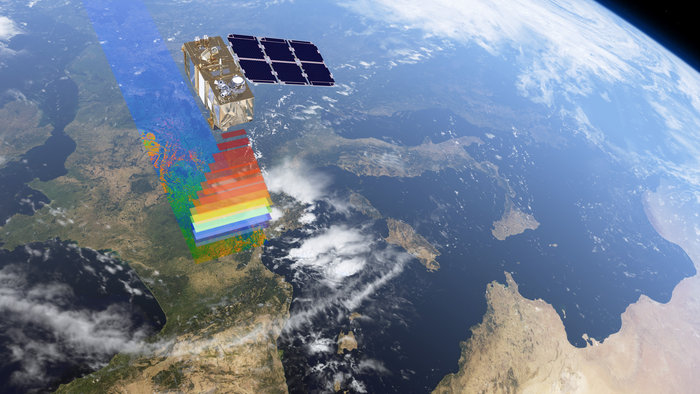
Methods
Basic Principles
Sunlight is a spectrum of radiation from infrared, through visible, to ultraviolet. It is filtered by the atmosphere and ocean, some wavelengths being absorbed and scattered, and some reflected back through the ocean and atmosphere to the satellite sensor. The sensor is multispectral, it measures reflected sunlight at specific wavelengths, or "bands", typically RGB, which are combined to create a "true colour" image, but also at wavelengths that can be processed to provide a range of Earth Observation data: such as the amount of sediment or plankton chlorophyll in the water, or on land the amount and health of vegetation or classification of land use.
For satellite-derived bathymetry, the essential principle is that there is a relationship between the amount & type of reflected energy detected by the sensor and the depth of water. This relationship is limited to 20-30 metres, the depth of light penetration in water, needs to consider the bottom type, since sand reflects more than, for instance, seagrass, and can be impossible in areas with high sediment load or algal blooms. But, if you can see the bottom from space it is possible to calculate the sea depth.
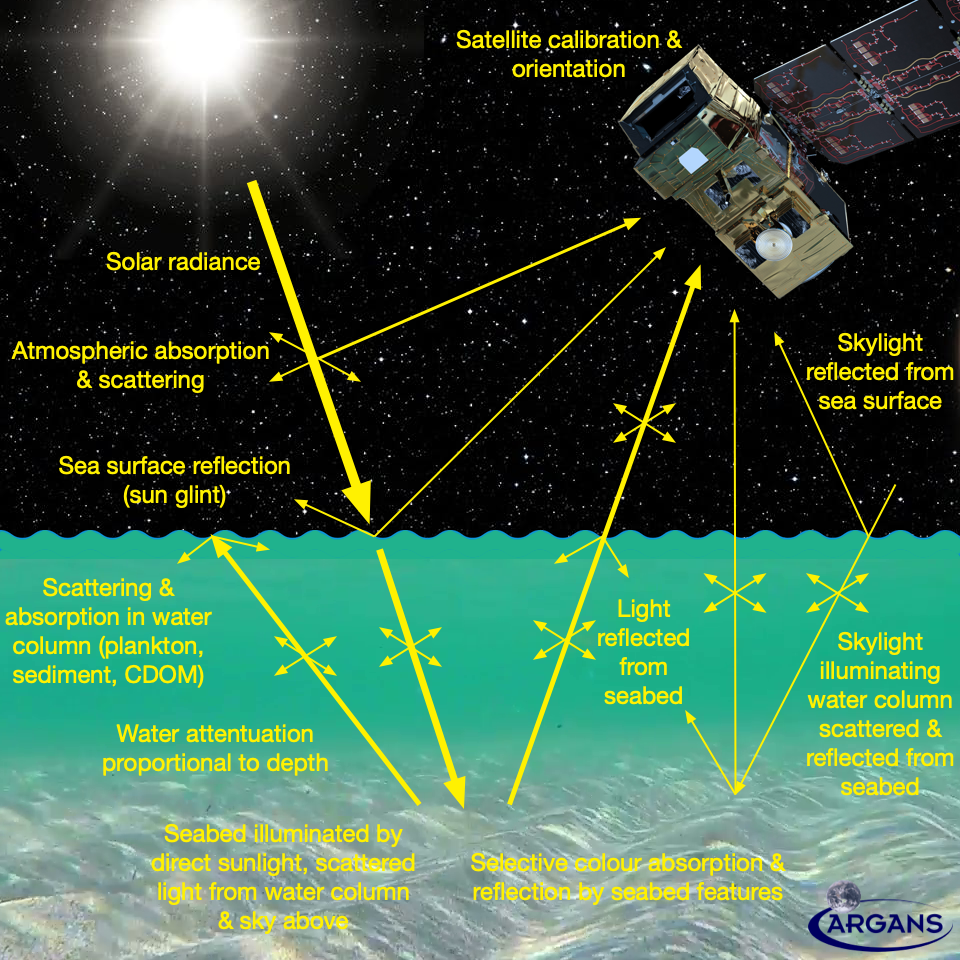
Algorithms | How it Works
Satellite-based bathymetry uses a processing chain comprising distinct algorithms:
Image selection is an important first step and images with high suspended sediment load or plumes, extreme ocean sun glint or high cloud cover are excluded due to negative effect these have on SDB
Spatial Registration
Using multiple images and/or images from differing sensors requires that image sequences are spatially aligned.
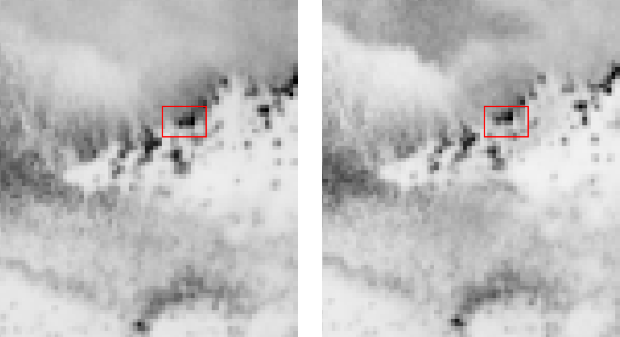
Land, cloud & white cap mask
For many of the processing steps it is advantageous to exclude pixels of land, clouds, cloud shadows and breaking waves (white caps).
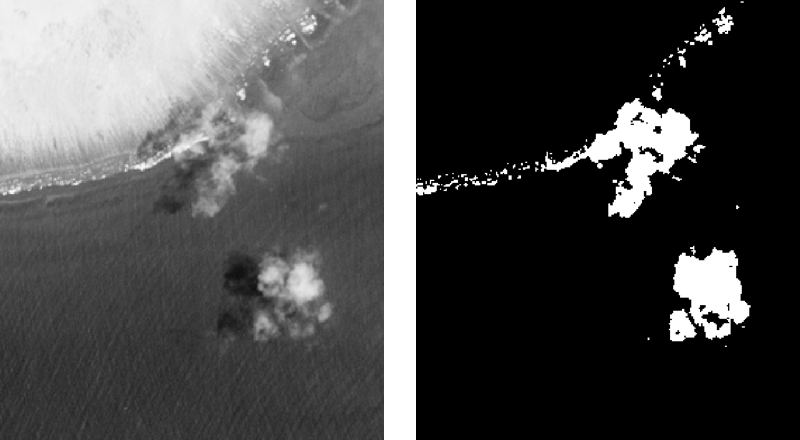
Sun Glint Removal
De-glint is a pre-processing algorithm aimed at removing the direct reflection of the sun from the air-water interface or ‘sun glint’.
Landsat 8
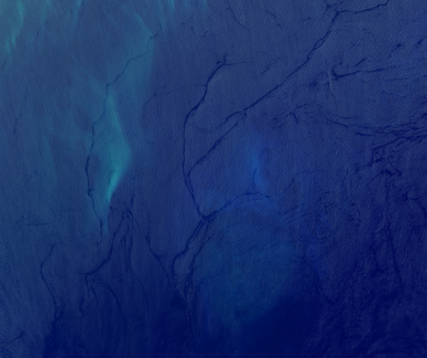
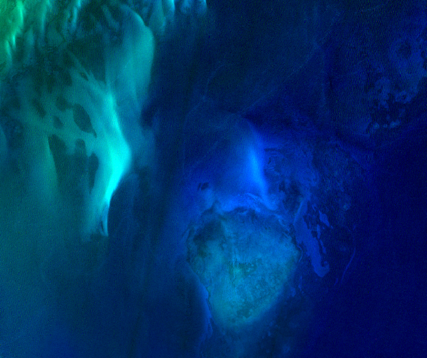
before de-glint | after de-glint
Sentinel-2
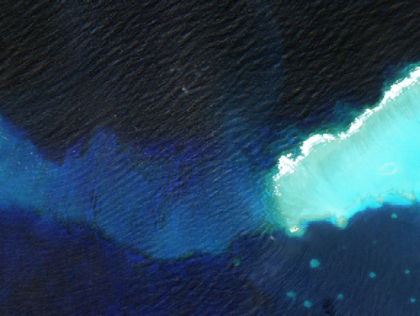
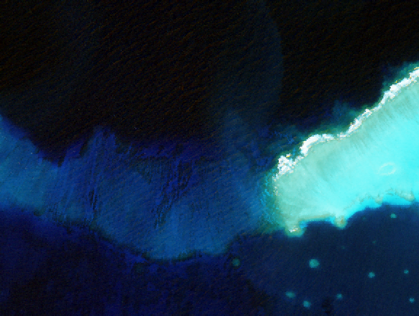
before de-glint | after de-glint
Atmospheric Correction
Subsequent to glint removal the next step it atmospheric correction i.e. the process of removing the effects of the atmosphere on the reflectance values of the image taken by the satellite sensor. Gases and aerosols in the atmosphere absorb and scatter radiation both when solar radiance is passing through the atmosphere and reflectance passing back out. The Sentinel-2 Sen2Cor SNAP module configured for maritime aerosols was used.
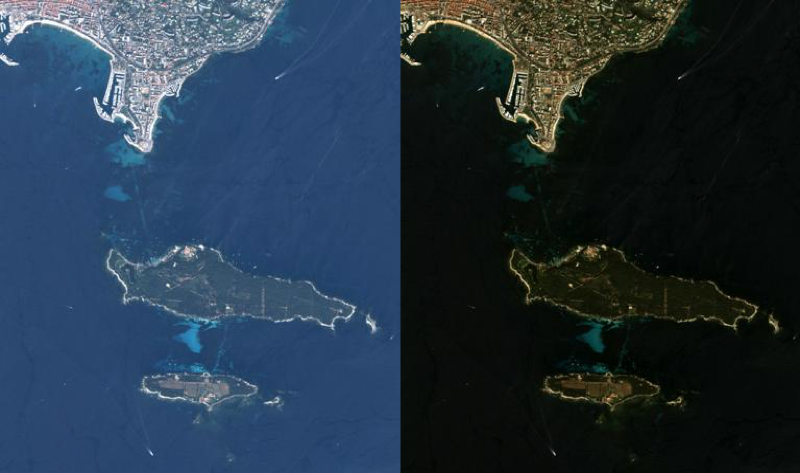
Atmospheric correction is difficult because 80-90% of the sensor signal in the blue-green is contributed by the atmosphere and the atmospheric path signal varies significantly. Over coastal zone images it is particularly problematic as a common assumption, that the open ocean is fully absorbent in NIR is invalid in turbid water and, in addition, coastal zone aerosols have contributions from both continental and maritime sources.
It is true to state that improvements in coastal zone atmospheric correction models would provide the most significant reduction of uncertainty in satellite derived bathymetry.
Depth Invariant Indices
Calculation of depth invariant indices is a water column correction step that is frequently a useful pre-processing step for benthic classification. Attenuation of the reflectance is approximately inverse exponential with water depth, therefore the transform, \[X_{i} = \ln\left(R_{i}{-R_{i}^{deep}}\right)\]approximately linearises the effect of depth on reflectance. \(R_{i}\) is the pixel reflectance in band \(i\), and \(R_{i}^{deep}\), is the deep water reflectance in that band.
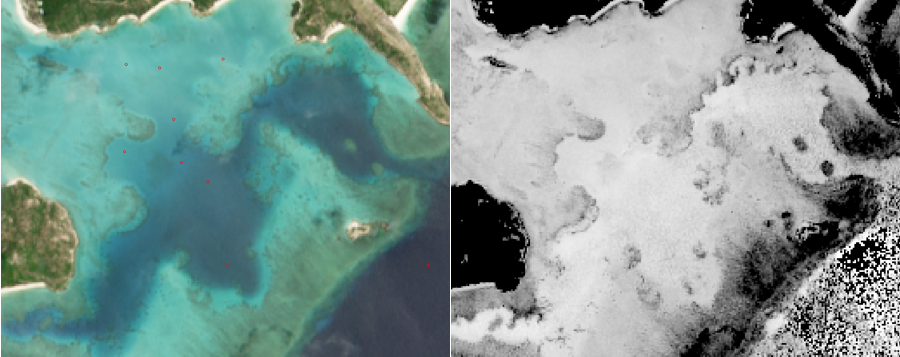
Habitat Mapping
Habitat classification is a complementary output available from satellite data bathymetric surveys. Due to the submerged nature of the marine habitats, classification requires pre-processing techniques, common to satellite derived bathymetry, for the removal of surface glint and the effects of variable depth e.g. water attenuation effects.
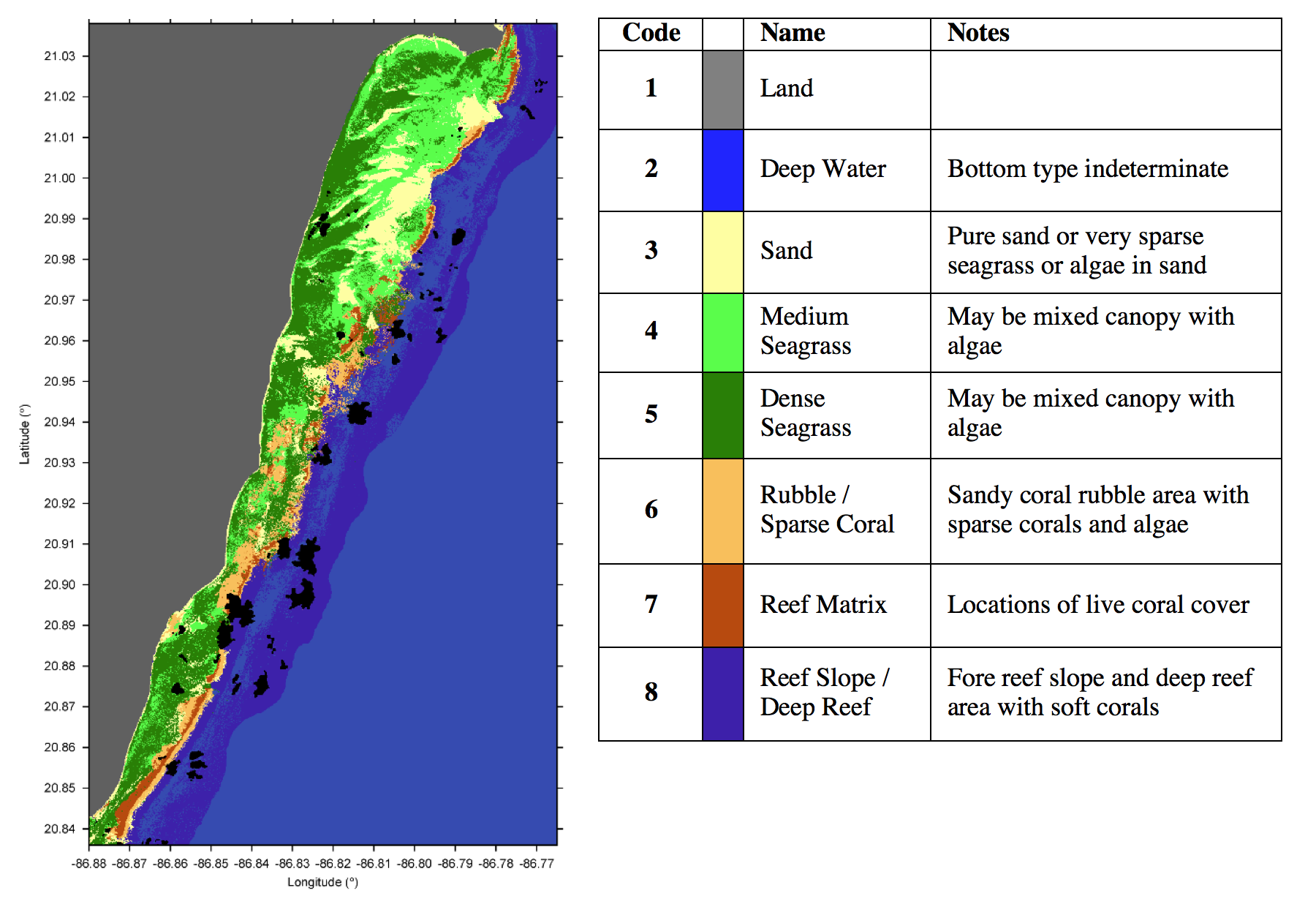
Benthic habitat classes used for high spatial resolution bottom type classification.
Physics Based Inversion
The main recent development in satellite derived bathymetry algorithms is that of physics-based model inversion approaches. These methods work by establishing a forward model for the spectral water-leaving remote sensing reflectance, \(R_{rs}(\lambda)\), based on the depth, optical properties of the water and bottom type. The majority of methods are based on a set of equations derived by Lee et al (1998; 1999) which can be represented by a function, \[R_{rs}(\lambda) \approx f\left({P,G,X,H,R(\lambda),\lambda}\right)\]Where \(P\),\(G\),\(X\) represent the amount of phytoplankton, dissolved organic matter (CDOM) and particulate backscatter, \(H\) is the depth and \(R(\lambda)\) is the bottom type reflectance – which can be taken from a spectral library or linear mixtures thereof..
IHO Nautical Charting
The ultimate objective in satellite derived bathymetric surveys is that the depth estimates, sub-surface features and, importantly, the error estimates, are sufficiently accurate and acceptable for maritime navigation and are used to create nautical charts that conform to the strict standards of the International Hydrological Organisation (IHO).
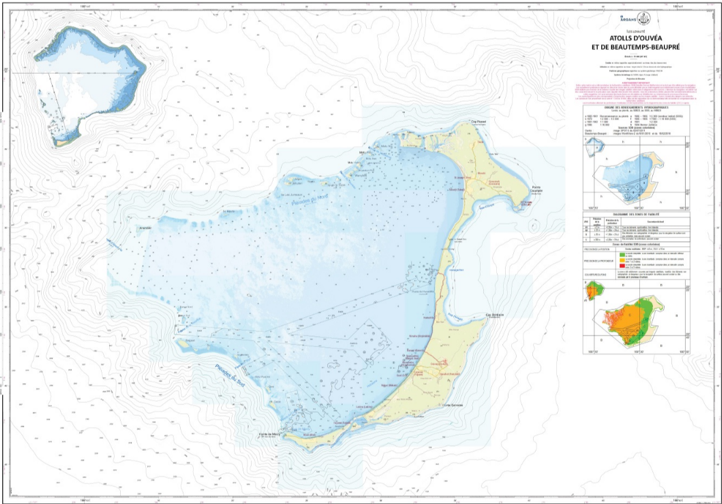
First nautical chart produced using SDB (SPOT-1) by SHOM of Ouvea in 1988
Test Sites
Puerto Morelos
Quintana Roo, Mexico
The region from Punta Nizuc, South of Cancun, to Puerto Morelos, provides a test area whereby a satellite-derived bathymetry demonstrator could be compared against a recent KOMSAT nautical chart.
Lampi Island
Mergui Archipelago, Myanmar
Lampi Island, is an island in the Mergui Archipelago, Mayanmar. The is no recent IHO nautical chart but there is an 1880 chart and that was used to calibrate the satellite derived bathymetry in a similar way as the use of the IHO chart for Puerto Morelos, although with less certain degree of the accuracy.
Nosy Be
Madagascar
The island of Nosy Be, off the Northwestern coast of Madagascar is the test site for a fully automatic processing of satellite imagery to derive bathymetry. A promising future improvement will employ machine learning.
Coral Harbour
Southampton Island, Canada
Coral Harbour is a small Inuit community located on Southampton Island, in the Nunavut territory (formerly part of the Northwest Territory), Canada. Fossilised coral found around offshore account for its name. The purpose in selecting this test site is to experiment how the bathymetry algorithms perform in sub-arctic waters.
FAQ
Do you need to use costly commercial VHR satellite images?
Actually, not really necessary. In comparison testing with two solutions: "good-enough" using 10m Sentinel-2 images and "best-achievable" using 2m VHR images it was discovered that the "good-enough" method yielded comparable results to the "best-achievable" method with a cost saving of €20 per square kilometre. Now we concentrate on using Sentinel-2 images and only resort to VHR for making comparative studies.
What happens if my survey site is usually cloudy or the water turbid?
One of the significant innovations that we have introduced is instead of using single images we use a stack of images. Previous research projects required a perfect cloudless image that would be the best condition possible to produce SDB. ARGANS takes a series of SDB models and uses a statistical approach, based on the exceptional signal / noise ratio of Sentinel-2 images to combine these into one result and therefore producing the ‘perfect model’ from a large database. By using multiple satellite images, errors within the image can be removed. This includes sediment plumes, effects of turbid water and human artefacts such as boats.